Einstein’s general theory of relativity predicts that each spinning body carries the fabric of space-time with it. This is referred to as “frame-dragging”.
In normal life, frame-dragging is both undetectable and insignificant since the effect is so little. Detecting the frame-dragging generated by the entire Earth’s rotation necessitates the employment of satellites such as the US$750 million Gravity Probe B, as well as the measurement of angular shifts in gyroscopes corresponding to around one degree every 100,000 years.
Fortunately for us, the Universe offers numerous naturally occurring gravitational laboratories where physicists can study Einstein’s predictions in action in great detail.
Our team’s study, published in Science, finds evidence of frame-dragging on a far larger scale, utilizing a radio telescope and a unique pair of tiny stars racing around each other at dizzying speeds.
The motion of these stars would have baffled scientists in Newton’s time, as they plainly travel in a twisted space-time, necessitating Einstein’s general theory of relativity to explain their paths.
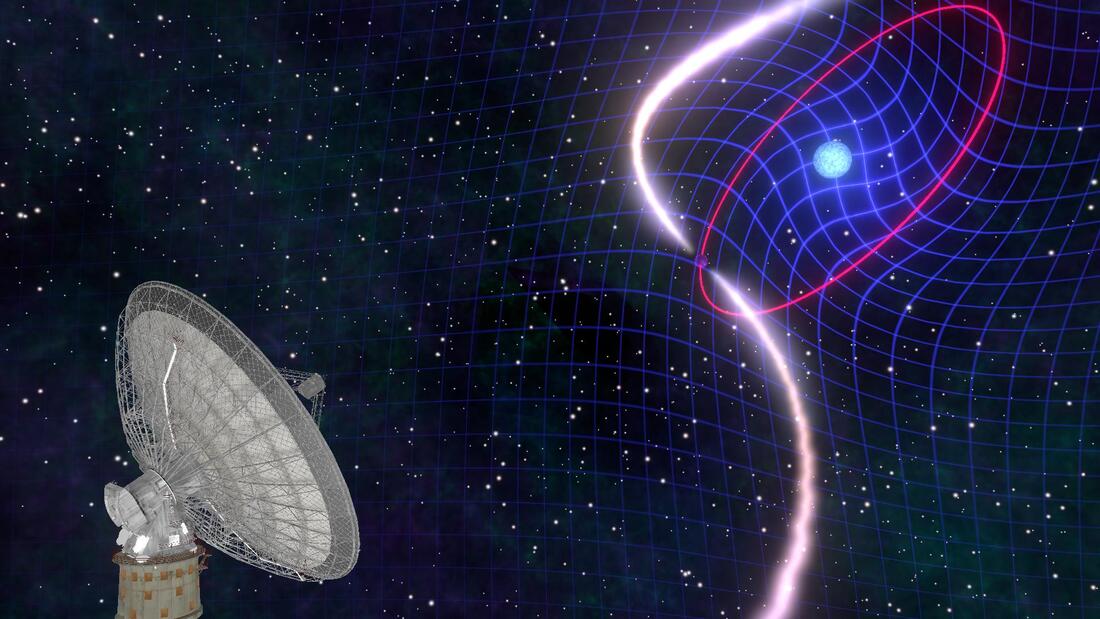
General relativity is the basis for current gravitational theory. It describes the exact motion of stars, planets, and satellites, as well as the flow of time. One of its lesser-known predictions is that spinning bodies move space-time along with them. The faster an object rotates and the larger it is, the stronger the pull.
This is especially important for white dwarfs. These are the remnant cores of dead stars that were once many times the mass of our Sun but have now depleted their hydrogen fuel.
What remains is equivalent in size to Earth, but hundreds of thousands of times larger. White dwarfs may also rotate very quickly, every minute or two, as opposed to once every 24 hours like Earth.
The frame-dragging induced by such a white dwarf would be around 100 million times stronger than Earth’s.
That is all well and good, but we cannot travel to a white dwarf and launch satellites from it. Fortunately, nature is nice to astronomers and has its own way of allowing us to study them: circling stars known as pulsars.
Twenty years ago, the CSIRO’s Parkes radio telescope found an unusual star pair made up of a white dwarf (about the size of Earth but 300,000 times heavier) and a radio pulsar (the size of a city but 400,000 times heavier).
Pulsars are on a whole other level than white dwarfs. They are composed of neutrons packed densely together rather than normal atoms, resulting in a very dense material. Furthermore, the pulsar under examination rotates 150 times every minute.
This means that 150 times every minute, a “lighthouse beam” of radio waves released by this pulsar passes by our location on Earth. We can use this to trace the pulsar’s orbit around the white dwarf by measuring when its pulse arrives at our telescope and knowing the speed of light. This approach demonstrated that the two stars orbit each other in less than 5 hours.
This pair, formally designated as PSR J1141-6545, is a perfect gravitational laboratory. Since 2001, we have been to Parkes multiple times per year to record this system’s orbit, which shows a wide range of Einsteinian gravitational effects.
Mapping the development of orbits is a time-consuming task, but our data are incredibly exact. Although PSR J1141-6545 is several hundred quadrillion miles away (a quadrillion is a million billion), we know it rotates 2.5387230404 times per second and has a tumbling orbit in space.
This suggests that its orbital plane is slowly rotating rather than fixed.
How did this system develop?
When two stars are created, the more massive one dies first, typically resulting in a white dwarf. Before dying, the second star sends matter to its white dwarf partner.
As this material descends towards the white dwarf, it creates a disk that revs up the white dwarf over tens of thousands of years, eventually rotating every few minutes.
In rare circumstances like this one, the second star might explode in a supernova, leaving behind a pulsar. The fast spinning white dwarf drags space-time with it, tilting the pulsar’s orbital plane. This tilting is what we discovered during our painstaking charting of the pulsar orbit.
Einstein himself believed that many of his predictions regarding space and time would never be seen. However, the last few years have witnessed a revolution in extreme astrophysics, with the finding of gravitational waves and the imaging of a black hole shadow using a global network of telescopes. These discoveries were achieved using multibillion-dollar facilities.
Fortunately, 50-year-old radio telescopes like the one at Parkes continue to play a part in general relativity research, as do patient efforts led by generations of graduate students.